Identify:
Heart rate measurements are a very versatile and useful tool for both starting and veteran athletes. Among many other uses [1], it can be used as a general gauge of how hard you are working out at that moment, and it can help determine improvement as your max heart rate increases. But putting a hand to your chest and counting the beats per minute is an impractical way to measure heart rate. So to help us measure this useful metric, we have heart rate monitors that can take our heart rate for us.
On the market, there are two types of heart rate monitors: Chest-strap monitors and wrist-strap monitors. Most people who aren’t even athletes probably have a wearable that can monitor heartbeats. Even most modern phones have the ability to measure your heart beats. However, despite their ubiquity, they have one major downfall: Wrist-strap monitors simply aren’t incredibly accurate. Besides the point of location, the wrist has several different factors [2] that make it difficult to get a good reading, ranging from skin-tone to motion. All of these factors produce noise that helps to muddy the results and can produce odd and inaccurate readings. To ensure we get the most accurate results to accurately determine our exercise maximum, it is this noise that we must turn to curb. But how do we go about reducing noise?
Formulate:
Modern wearable HRMs use a technology called photoplethysmography or PPG. In essence [3], the device shines a light through a bulb (usually an LED), that passes through the skin and muscles. A majority of this light will be absorbed by the surrounding skin and muscles. The blood absorbs the light that does make it through, which is the crux. By measuring the amount of light that is absorbed, the device can pick up on pulses in the blood based upon the differences in these absorbances. This technology is very similar to how spectrophotometers work, with both measuring the absorbance that a material has. But unlike a spectrophotometer which measures the absorbance through the material, PPG measures constantly, listening in for very small changes in absorbance that can be used to determine heart rate.
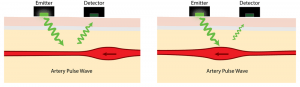
An example of PPG. Pulses within the vessel cause even more light to be absorbed than would normally. This is what the wearable is measuring.
PPG is very sensitive to physical sensations like bumps and pumps, which produces noise. When the sensor is jostled due to motion, the small amount of signal that is recorded gets muddled, which produces the majority of the noise that we are trying to cut down. This noise can cause very odd results, such as heart rates that vary wildly [4]. The noise is not present while not in motion, but for athletes who want to know how high their heart rate can get, this is not acceptable. As noise caused by motion is the leading cause of these very odd discrepancies in heart rate, we need to lower it as much as possible.
In order to solve this, we are going to separate the signals read between three metrics: a DC, an AC, and a noise component. We shall be ignoring noise caused by the optical signal, as it is subtracted by the sensor through the use of ambient light measurements [5]. Thus, the incoming current that is read can be simplified as:
Incoming Current = DC component + AC component + Noise
The DC component of the signal comes from changes in respiration in the vessel, while the AC component comes from variation in blood volume due to the heartbeat.
Solve:
If we want to reduce the amount of noise, we are going to have to pass the incoming signal through a filter. When the signal comes back to the wearable, it must interpret the signal, from raw absorbance values to electrical signals that are then interpreted by the wearable. And since we assume that optical light noise is negligible, we can focus on removing noise due to motion. Using biophysical-signal characterization techniques [6] as our filter, we can compare the incoming signal, and remove noise caused by motion. The remaining non-noise components will have to be amplified in order to get a complete, accurate signal. We do this in order to fill in the gaps made when we remove several points of noisy data.
By using a current filter, we hope to minimize the amount of noise that is detected alongside the heart rate of the monitor. If we can eliminate the noise read alongside the signal, we can get more accurate results, as the signal interpreted would consist only of the AC and DC components. However, this approach focuses only on noise made by motion. As stated before, there are several different sources of noise that interfere with accurate measurements. While motion is one of the primary sources of noise, other factors like skin tone, gaps between the sensor and the skin, and the location of the sensor can introduce more noise. This solution also has the possibility of cutting off accurate readings that are interpreted by the sensor as noise. Athletes who do a lot of vigorous exercises may find that their heart rates are inaccurate under this solution if their heart rates spike hard enough during exercise.
[1]: Shmerling R. How’s your heart rate and why it matters? – Harvard Health. August. https://www.health.harvard.edu/heart-health/hows-your-heart-rate-and-why-it-matters. Published 2017.
[2]: LeBeouf S. Five Challenges of Optical Heart Rate Monitoring. Sensors online. https://www.sensorsmag.com/components/five-challenges-optical-heart-rate-monitoring. Published 2016.
[3]: Cheriyedath S. Photoplethysmography (PPG). News Medical Life Sciences. https://www.news-medical.net/health/Photoplethysmography-(PPG).aspx. Published 2016.
[4]: Oniani, Salome & Woolley, Sandra & Pires, Ivan & Garcia, Nuno & Collins, Tim & Ledger, Sean & Pandyan, Anand. (2018). Reliability Assessment of New and Updated Consumer-Grade Activity and Heart Rate Monitors. 10.13140/RG.2.2.35628.72328.
[5]: Wearables | OSRAM. https://www.osram.com/os/applications/mobile-competence/mobile_competence_wearables.jsp.
[6]: Maity S, He M, Nath M, Das D, Chatterjee B, Sen S. BioPhysical Modeling, Characterization and Optimization of Electro-Quasistatic Human Body Communication. IEEE Transactions on Biomedical Engineering. http://arxiv.org/abs/1805.05200. Published May 14, 2018.