
RESEARCH TOPICS
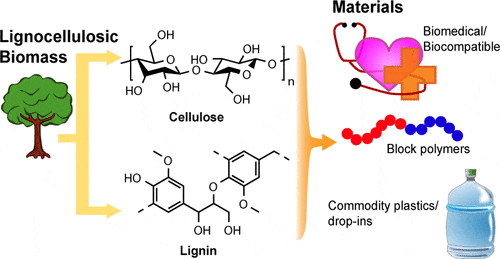
An area of emphasis in the Epps group is the synthesis of functionalized monomers from these renewable feedstocks, with a particular focus on lignin-derived compounds. Lignin can be depolymerized into its building blocks, which can then be modified to create monomers for the synthesis of new materials through controlled radical, ring-opening, anionic, and cationic polymerizations. Additionally, inherent functionalities on lignin aromatics can be helpful to mitigate the toxicity concerns of conventional aromatics. Of particular interest to our group is developing structure-property relationships of these materials and investigating ways to valorize biomass feedstocks such as making biobased monomers for 3D printing, generating lignin-derived steric stabilizers for fabrication of sustainable MOF/polymer composites, and using bio-based catalysts for synthesizing components of sustainable thermoset-forming systems.
Lithium-ion batteries are a rapidly-growing industry with widespread applications and superior performance in comparison to other energy storage devices. Block polymer electrolytes show promise in addressing some of the safety and performance limitations of the current liquid electrolytes in lithium-ion batteries. We are interested in designing block polymer electrolytes that can transport lithium ions efficiently to reduce charging times, have sufficient mechanical strength to reduce lithium dendrite growth that can cause short-circuiting between electrodes, and are processible via inexpensive means.
Tapered block polymers are block polymers with interfacial regions that taper from one polymer block to another polymer block (in a well-defined fashion over a well-defined region of the copolymer). These materials are of high interest because the tuning of monomer sequence gives rise to higher ionic conductivities, favorable nanostructures with well-connected ion-conducting pathways, and improved processibilities in comparison to non-tapered analogues while maintaining the desired chemistry and mechanical properties.
Block polymer/homopolymer blends also present an opportunity to improve ionic conductivity and processibility. The appropriate combination of polymer molecular weights enables the formation of homopolymer-rich channels that act as “superhighways” for lithium ions.
The precise quantification of structural characteristics of the block polymer electrolytes allows us to identify key parameters that govern the material properties. Experimental techniques such as reflectometry provide nanometer-level resolution to ascertain distributions of monomer segments and ions, while computational modeling suggests mechanistic insights into block polymer assembly. This understanding allows us to advance our material designs efficiently.
The incorporation of porous nanoparticles into polymers to form mixed matrix and fiber-based composites is a burgeoning area relevant in applications such as separations, catalysis, drug delivery, and protection. In particular, metal-organic frameworks (MOFs) are highly tunable materials with extraordinarily high surface areas. Adding MOFs to polymers, which also are highly tunable, offers advantages over other systems.
Polymer-based composites fabricated with MOFs have inherent advantages over traditional composites because of the partially organic nature of MOFs; however, shortcomings still exist, such as agglomeration of MOF crystals, defects at the MOF-polymer interface, and a lack of systematic configurations (e.g., arrays) of MOFs within polymers. We are interested in utilizing lignin-derived polymers as steric stabilizers for metal organic frameworks (MOFs) and process them into nanofiber composites via electrospinning to attain comparable or better composite properties as that of the petroleum-derived polymeric counterparts.
Engineering of the MOF-polymer interface can be achieved through altering the MOF crystal as well as changing processing conditions used to fabrication composites. In the former, some of the organic linkers on some MOFs can be modified using acyl chloride chemistry to impart functional groups with similar chemical makeup to the polymer, which results in reduced defects as well as better dispersion of MOF crystals in both film and fiber forms.
Techniques used to mix preformed MOFs with polymers leads to active materials for several applications; however, these composites often suffer from particle agglomeration that occurs during processing of the composite. An alternative strategy is to seed polymers with MOF precursor salts and then grow crystals after polymer processing has occurred. Changing the metal type, salt anion, and concentration affects solution viscosity and conductivity, which translates to changes in processing. Metals dispersed within the fabricated composites act as nucleation centers for in situ growth of MOFs.
Many applications and devices require controlled distribution of material functionality in multiple dimensions. At the nanometer length scale, attempts to meet this challenge have included template-mediated materials chemistry. Interest in block copolymers has evolved because of their potential use in numerous nanotechnologies including nanotemplating, filtration membranes, and organic optoelectronics (LEDs and photovoltaics). Self-assembly of block copolymers in thin films is a complex phenomenon. A large parameter space, including film thickness, annealing conditions (thermal or solvent), molecular mass, and surface energy, governs the film morphology. Surface energetics and interface interactions also direct morphology orientation.
The behavior of thermally-responsive block copolymers compounds this complexity. When a thermally-responsive block copolymer undergoes a thermal transition resulting in a mass loss, the parameter space expands to include volume fraction shift, thickness decrease, surface energetic shifts of the relative blocks, and a change in substrate and free surface energetics. The resulting phenomenon is impacted by the complexity of multiple and often co-dependent variables. Control in chemically amplified transformations such as in thermal deprotection reactions can prove extremely useful especially when the self-assembly of the block copolymer is affected. Current investigations include controlling the final self-assembled morphology and orientation of thermally-responsive block copolymers using different surface chemistries and fabrication techniques as well as high-throughput methods for rapid characterization and identification of critical parameters.
An important aspect of exploiting high-throughput methods has been the development of novel gradient fabrication devices to efficiently probe the effects of substrate surface energy/chemistry and annealing conditions on block copolymer thin film morphology. These gradient approaches are becoming increasingly important for mapping the phase behavior of new materials for specific applications. In the following example, we used controlled vapor deposition to generate a gradient in substrate surface energy/chemistry and we show how the orientation of a cylinder-forming PS-b-PMMA thin film evolves with changes in substrate surface chemistry from a pure benzyl silane monolayer on silicon (left) to a pure methacryl silane monolayer on silicon (right), with gradient compositions and morphologies shown in between.
We have also designed a solvent resistant microfluidic mixing device that produces discrete gradients in solvent vapor composition and/or concentration to quickly and easily examine the use of solvent mixtures (versus a single solvent) for controlling thin film self-assembly. The image below shows a schematic of our solvent vapor annealing setup with the microfluidic device and its use as a screening tool to locate phase transformations in a poly(styrene-b-isoprene-b-styrene) triblock copolymer as a function of solvent composition and swollen film thickness.
Polymeric nanomaterial assemblies have several attractive features including tunability, control over the size and structure of the assemblies, and enhanced stability. Furthermore, the chemical versatility of polymers enables the incorporation of various stimuli-responsive moieties. Our group is interested in exploiting these valuable properties to develop polymer assemblies, which could be utilized in a broad range of applications.We are currently focusing on biomedical aspects such as nucleic acid and small molecule drug delivery. Controlled release of these therapeutics is widely recognized as one of the most significant challenges hindering clinical success. To this end, our lab has designed and synthesized novel photo-responsive block copolymers to bind and encapsulate nucleic acids and mediate efficient release upon application of the photo-stimulus. We have implemented a variety of strategies to expand the versatility of our platform, including formation of mixed polymer carriers to tune the amount of siRNA released, incorporation of anionic excipients for theranostics, and development of a hybrid lipid-polymer formulation to efficiently transfect human primary cells. Furthermore, elucidation of the mechanisms that underlie the gene silencing process has facilitated the design of a simple mathematical model to predict the kinetics of siRNA-mediated gene silencing.